Self-Circulating/-Regulating Microreactor for On-Chip Gas Generation from Liquid Reactants
Dr. Likun Zhu, Mechanical Engineering, NSF Award # 1264549.
Dr. Likun Zhu, Mechanical Engineering, NSF Award # 1264549.
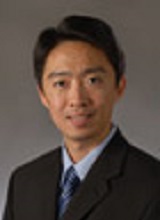
Generation and handling of gaseous species with reduced parasitic power consumption and parasitic mass has been a growing challenge in many types of chemical reactors, including micro power sources[1], gas-liquid synthesis [2], micro flame ionization detector [3,4], solar water splitting system [5,6], and microbial electrolysis cells [7-9]. To confront such a challenge, a novel self-circulation, self-regulation mechanism is proposed to generate gaseous species from liquid reactants on demand [10]. This project seeks to understand the process control and dynamics of an integrated microfluidic gas generator with self-circulation and self-regulation functionalities. To achieve this objective, catalytic decomposition of hydrogen peroxide is employed as a basic model system to perform fundamental studies. The dynamics of bubble-driven liquid circulation, self-regulation, mechanism of gas/liquid separation, and reactant utilization are investigated systematically to gain knowledge regarding the interplay of various factors in the microfluidic reactor and associated phenomena.
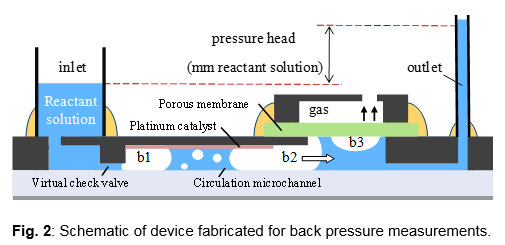
In this REU summer project, the student will conduct experiments to investigate the gas generation rate with different reaction channel dimensions. The dimensions of channels will determine the backpressure and the capillary force to pump the reactant solution. Increasing the channel dimension will decrease the maximum backpressure and the capillary force for pumping. However, large channel dimension will result in large gas volume for each pumping cycle because the chemical reaction rate is much faster than liquid pumping rate. A setup shown in Figure 2 will be constructed to measure the gas generation rate and reactant solution flow rate at different back pressures with different channel dimensions. The liquid height at the outlet tubing will be measured as a function of time and recorded with a video camera. Gas generation rate will be measured by a gas flow meter and reactant solution flow rate can be measured by tracking the movement of the liquid height at the outlet tubing. The height of the liquid column at the outlet tubing (small diameter) will be recorded with a digital camcorder and measured as a function of time. Since a much larger tubing is used as the inlet reservoir (large diameter), the inlet height of the solution is assumed to be constant. Therefore, the difference in liquid heights is a measure of the maximum pumping head in units of pressure. The velocity at which the meniscus moves at the top of the outlet is a measure of the pumping rate. If carried out successfully, the proposed work is expected to establish a correlation among channel dimension, back pressure and liquid pumping rate. This project includes mechanical design and manufacturing for the micro/nanofluidic devices and gas generation testing/analysis. This research project can provide the undergraduate student a good opportunity to conduct research work in a lab setting using basic principles that he/she have learned in class. After completing this project, the undergraduate student will learn to solve complex multidisciplinary problems at a level normally undertaken by graduate students or professional engineers. This will prepare him/her to be a leader in engineering analysis.
References:
N. Kroodsma, L. Zhu, J. Yeom, M. A. Shannon, and D. D. Meng, "A Fully-Enclosed Micro PEM Fuel Cell with Self-Regulated Fuel Delivery and Shut-Down," PowerMEMS, Washington DC, USA, 9-12, 2009.
C. P. Park and D. P. Kim, "Dual-Channel Microreactor for Gas-Liquid Syntheses," Journal of the American Chemical Society, 132, 10102-10106, 2010.
C. H. Deng, X. H. Yang, N. Li, Y. Huang, and X. M. Zhang, "A novel miniaturized flame ionization detector for portable gas chromatography," Journal of Chromatographic Science, 43, 355-357, 2005.
S. Zimmermann, P. Krippner, A. Vogel, and J. Muller, "Miniaturized flame ionization detector for gas chromatography," Sensors and Actuators B-Chemical, 83, 285-289, 2002.
E. Selli, G.L. Chiarello, E. Quartarone, P. Mustarelli, I. Rossetti, and L. Forni, "A photocatalytic water splitting device for separate hydrogen and oxygen evolution," Chemical Communications, 5022-5024, 2007.
M. G. Walter, E. L. Warren, J. R. McKone, S. W. Boettcher, Q. Mi, E. A. Santori, and N. S. Lewis, "Solar Water Splitting Cells," Chemical Reviews, 110, 6446-6473, 2010.
J. Ditzig, H. Liu, and B. E. Logan, "Production of hydrogen from domestic wastewater using a bioelectrochemically assisted microbial reactor (BEAMR)," International Journal of Hydrogen Energy, 32, 2296-2304, 2007.
B. E. Logan, D. Call, S. Cheng, H. V. M. Hamelers, T. H. J. A. Sleutels, A. W. Jeremiasse, and R. A. Rozendal, "Microbial Electrolysis Cells for High Yield Hydrogen Gas Production from Organic Matter," Environmental Science & Technology, 42, 8630-8640, 2008.
B. E. Logan, "Scaling up microbial fuel cells and other bioelectrochemical systems," Applied Microbiology and Biotechnology, 85, 1665-1671, 2010.
L. Zhu, N. Kroodsma, J. Yeom, J. L. Haan, M. A. Shannon, and D. D. Meng, "An On-Demand Microfluidic Hydrogen Generator with Self-Regulated Gas Generation and Self-Circulated Reactant Exchange with a Rechargeable Reservoir," Microfluidics and Nanofluidics, 12, 735-749, 2011.